The Science of Plant Diseases
- sherbornesciencecafe
- Oct 4, 2021
- 8 min read
Geoff Dixon
21/09/2021
⦁ Introduction
In a resumption of its usual, pre-pandemic schedule, Science Cafe welcomed Professor Geoff Dixon, horticulturist, microbiologist (mainly of soil borne diseases), keen gardener, owner of GreenGene International (providing consultancy to Agricultural sectors) and member of School of Agriculture at Reading University, to speak about plant diseases. His presentation covered plant diseases in general and specifically, clubroot (Plasmodiophora brassicae) in brassicas as a case study. Plant science is hands-on, demanding great investigative powers in the laboratory and excellent practical skills in the field (hence requiring scientists to have ‘one foot in the furrow, one hand in the laboratory’)
Like diseases and epidemics that occur amongst faunal populations (think Covid), plant diseases are similar agents of opportunity, targeting a population of susceptible hosts large and vulnerable enough for the disease to get a firm hold. Notable diseases today in wild stocks are Ash Dieback Disease (Hymenoscyphus fraxineus), Dutch Elm Disease (ascomycete microfungi) and Sudden Oak Death (Phytophthora ramorum). Even casual gardeners are confronted with agents of infection. At this time of the year, black spot on roses (Diplocarpon rosae) and pear scab (Venturia pyrina) evident. Responses are initiated, in wild plants, through evolutionary change, but in agricultural crops, carefully husbanded by their human protectors, once an infective agent is identified, many more options of control are available.
Historically, in agricultural crops, the ravages of disease are nothing new. The Romans contended with rust on cereals; ergotism, until modern times, was not an infrequent intrusion into the life of agriculturists causing St Anthony’s Fire; and European vineyards were hit hugely by insect and mildews attacks in the 19th century (investigated by Louis Pasteur).
Causative agents of disease include fungi, viruses, bacteria, mycoplasmas and protists. The first three are familiar, mycoplasmas and protists less so. Mycoplasma-like organisms are parasitic and generally considered to be somewhere between bacteria and viruses in size, shape and function with a lack of both cell wall and nucleus. A protist is a eukaryotic organism that is not a plant, animal or fungus, probably sharing a common ancestor, though as protists are a grouping of convenience, some may be more related to animals, plants or fungi than to other protists. Examples include amoebas, Phytophthora (cause of the Irish potato famine) and slime moulds.
Being small, prolific opportunists and easy casual travellers, they can respond quickly to changing circumstances both in geographical range and extent of activity. Two or three decades ago, in the UK, fungal diseases predominated, whereas in more southern countries, bacterial diseases were the main cause for concern. Now, bacterial diseases are in the ascendancy in the UK, another significant marker of the pace and extent of climate change.
In a world where food supplies do not meet demand, over one third of crops are lost to pathogens, pests and environmental stress. The study of plant diseases is therefore critical to a sustainable agricultural sector.

Like human epidemiologists in their fight against Covid (or any other disease) plant pathologists must adopt a well-established approach in response to the appearance of new or resurgent diseases. This involves:
⦁ Describing the disease
⦁ Identifying the pathogen
⦁ Naming the pathogen
⦁ Identifying the environmental conditions for its proliferation
⦁ Understanding its capacity for spreading (epidemiology)
⦁ Effects on the host in terms of anatomy, physiology, and molecular biology
⦁ From the above determining control methods
A major difficult with plant pathogens is that as they proceed through their life cycle, they can, at various stages, appear like different diseases, hence the importance of precise laboratory and field work. Fungi, for example can propagate asexually, sexually or parasexually to name but one difficulty. To be consistent in identifying diseases, a standardised approach is required.
The protocol for this is known as Kock’s Postulates and is used to establish a causative relationship between a microbe and disease. It is named after the notable German physician and microbiologist, Robert Kock, discoverer of the specific causative agents of deadly infectious diseases including tuberculosis, cholera, and anthrax.
Kock’s approach involves extracting diseased tissue, cleaning, culturing on agar, determining the infective agent’s preference for nutrients, extracting, inoculating into healthy tissue and comparing disease symptoms. A seemingly straightforward approach but one that can take around 20 years to complete. Kock’s Postulates dates back to 1884 and whilst still a very valid approach, it has been updated by the Bradford Hill Criteria and genome sequencing.
2. Brassicas
Brassicas are an extremely important crop, secondary only to cereals and encompass, cabbages, turnips, mustards, watercress, nasturtiums to name but a few.
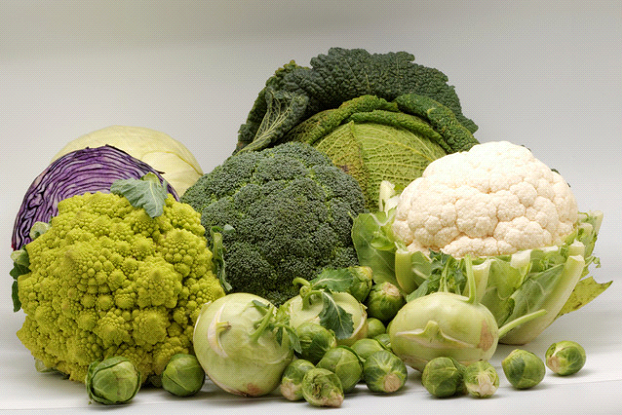
Globally, brassica crops provide the greatest diversity of agricultural products derived from a single genus. They deliver leaf, flower and root vegetables that are eaten fresh, cooked and processed; used as fodder and forage, contributing overwintering supplies for meat- and milk- producing domesticated animals; sources of protein and oil used in low fat edible products, for illumination and industrial lubricants; condiments such as mustard, herbs and other flavourings; and soil conditioners such as green manure and composting crops. Arabidopsis thaliana, another brassica, is a rather insignificant weed with little direct significance for agriculture but several traits make it an indispensibe model for understanding the genetic, cellular, and molecular biology of flowering plants. It was the first plant genome to be decoded.
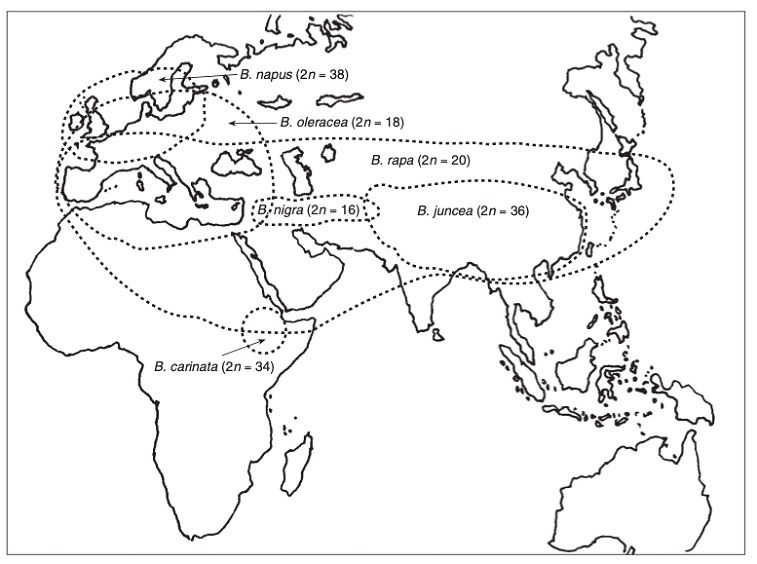
Historical investigation (the so-called Triangle of U) suggests that three ancestral species of brassica combined by hybridisation to create the three common vegetable and oilseed crops we know today.
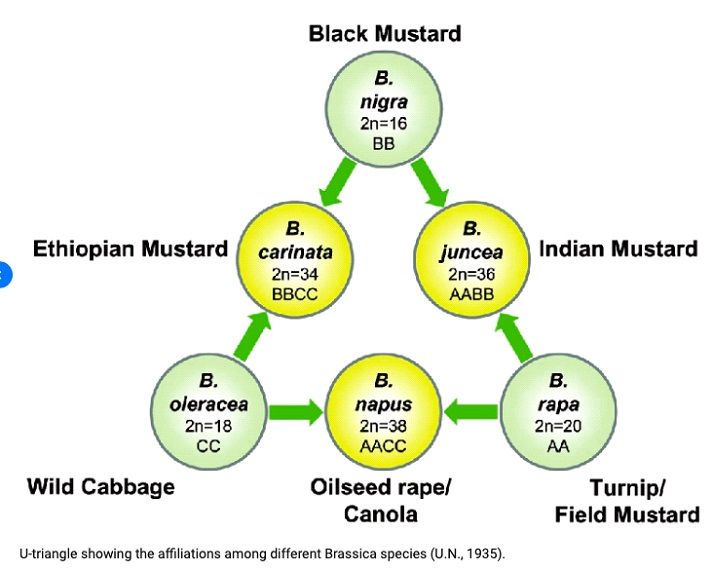
The "triangle of U" diagram, showing the genetic relationships among six species of the genus Brassica. Ancestral species lie at the corners of the triangle
3. Club Root (Plasmodiophora brassicae)
To illustrate the challenges of disease identification and management, Professor Dixon used the example of the protist, clubroot (Plasmodiophora brassicae) in brassicas. Reports of clubroot extend back to the 13th century. It was first described in detail in 1860 by Russian scientist Mikhail Voronin, in response to a severe outbreak in St Petersburg. In the Russian agricultural system, brassicas were critically important crops providing Summer food for animals and a stored crop to get the population through the severe winters.
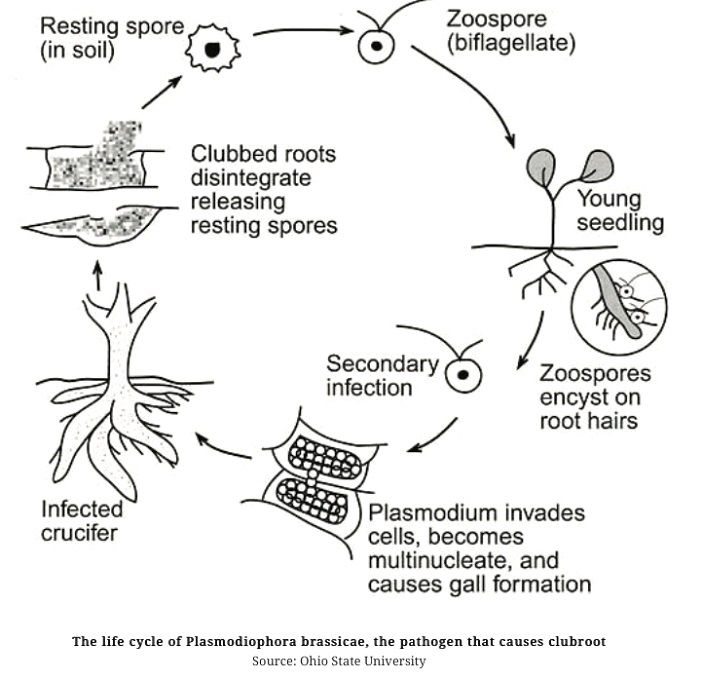
Resting spores germinate in Spring producing zoospores that swim in soil water to root hairs. Exudates from the host root sets off germination. After initial infection, the pathogen forms an amoeba-like cell. This multiplies and joins with others to produce a plasmodium, a mass of protoplasm with many nuclei which divides to produce many secondary zoospores which enter the soil. These second generation zoospores reinfect nearby plants, including the original host, invading the cortex of the root. The cells multiply and/or join others to form a secondary plasmodium. As it develops, host hormones are altered causing infected root cells to swell. These swellings form galls or clubs. Galls extract water and nutrients, depriving the above ground parts which die. After maturity, the secondary plasmodia divide into many millions of resting spores. P. brassicae has a life cycle period of ~30 days.
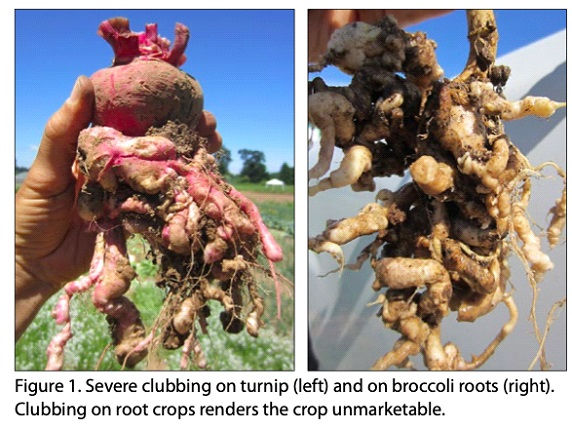
Symptoms of P. brassicae are first seen above ground as slight wilting and yellowing of lower older leaves. Later stunting occurs in new growth and the plant eventually dies. Roots are swollen (with the formation of galls), distorted, and malformed with a loss of finer root hairs. Host cells are disrupted, regulation destroyed, matched by an increase in host DNA/RNA, increase in host sugars, increase in growth regulators. P. brassicae is deficient in housekeeping genes, relying totally on the host brassica for this.
Interestingly, the nearest faunal relative to clubroot is Plasmodium falciparum, the cause of malaria in humans.
Besides its impact on essential agricultural crops, P. brassicae is a particular challenge for scientists. It produces lots of spores and is well adapted to its host. Resting spores are physically protected with five layers of chitin and long lived >18 years (half-life of ~3.6 years) meaning P. brassicae is unlikely to be eliminated from soil once established. The longevity of the resting spores is a crucial factor in contributing to the seriousness of the disease. It has a short free-living stage, a hidden and protected lifecycle within the host root. Even now mysteries remain- scientists are not sure where cell meiosis occurs. Besides the organism itself, there are added complexities to contemplate such as soil/organism interaction and environmental influences.
P. brassicae is easily spread, by animals, through their gut (think transferring livestock between different countries/regions), infected soil on boots or machinery, irrigation water, seed potatoes brought in from elsewhere, sheep, wool, faeces, compost, manure etc.
4. Tackling P. brassicae
There are no licensed chemicals that can be used to control P. brassicae, at least in Europe, due to detrimental environmental effects. The concept of Integrated Management is used to mitigate its effect. With no single action able to tackle the problem, many different strategies, each giving the plant a slight advantage are advocated, with the expectation that when combined, or integrated, the overall effect will be significant. Studies are monitored by controlled field experiments, field trials and trudging through farmers’ fields to make the necessary observations. The best defence against P. brassicae is to avoid getting it in the first place by protecting against its importation to unaffected areas.
Historically, early farmers will have observed that P. brassicae is a disease of acid, not alkaline, soils and will have noted that liming the soil, thereby increasing its pH (i.e. increasing alkalinity) will have controlled the ravages of the disease. Modern science indicates that a pH of 7.2-7.4 is probably ideal, any higher restricts micronutrients available, particularly manganese. For it to be immediately active, lime must be either CaO or Ca(OH)2, known as slaked lime. Ports along the coast of the southwest abound with now defunct lime kilns where limestone (brought in by ship) was burnt with coal to produce slaked lime for local farmers. In contrast to the active limes, powdered limestone, CaCO3, takes several months to become active in soils. Lime needs to be applied in several doses over the lifecycle of P. brassicae rather in one application and care must be taken to eradicate low pH microsites that will continue to harbour the disease. The suppressive nature of lime works by interfering with conversion from the first to the second plasmodium. More recently, the availability of the element Boron in the soils has been found to be of importance, more so, it is thought, than that of lime. It is highly soluble and easily washed out of the soil and requires regular applications every fortnight or so. Nitrate concentration too is of importance.
Crop rotation is also an essential suppressant. Spores have a half-life of ~3.6 years. Some growers suggest a rotation of 5-6 years will significantly reduce disease, whilst a rotation of 4-5 years might result in serious crop loss.
Developing genetic resistance is another effective way to proceed. Even without human interference, this approach has already been adopted by several brassicas. The so-called ‘Triangle of U’ (see above) shows how early brassicas swapped and exchanged genetic material to produce more robust strains. Unlike their faunal cousins, plants are much more permissive in exchanging genetic material to achieve evolutionary advantage.
Sources of useful resistance have been found in several brassica species. There is major single gene resistance in some turnips which gives good protection. It has the downside that if overwhelmed by genetic changes in P. brassicae, swathes of brassicas would be affected. An alternative approach is encouraging minor gene resistance whereby groups of genes fortuitously work together providing some useful resistance. Because several genes are involved it is more resilient to redundancy than major single gene resistance. There are commercial factors to deliberate when considering gene resistance. The Shetland Cabbage, an ancestral brassica habituated to the northern fringes of Europe, often grown for animals, produces useful minor gene resistance, though when crossed with conventional cabbages produces a very unattractive, unmarketable offering. However, making constant crosses, over many years, may produce an acceptable, resistant cabbage, though the complex, permissive nature of plant gene manipulation can mean cross-sterility genes are problematic. Molecular biology provides further inroads into developing resistance, identifying key enzymes and their associative genes and how these impact on the P. brassicae life cycle. Technology has also assisted. Drones can scan fields of growing brassicas to identify problem plants, and ground-based tractor machinery allows multisampling of soil such that soil variables can be observed in high spatial resolution across a field of interest.
5. Conclusion
The science of plant diseases provides a key approach in maintaining crop yields in a world where human population pressures mean that a significant proportion is malnourished. Climate change is happening a rate faster than for many tens of millions of years, yet plant diseases seem very adept at taking advantage of this. Whilst effective techniques exist to mitigate against infective agents, plant diseases are currently winning the war of attrition with scientists.
Comments